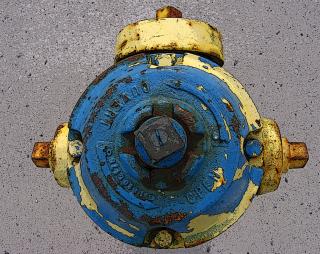
Carbon capture and storage (CCS) is a collection of technologies often mentioned in connection with global warming. Essentially, the idea is to capture the carbon dioxide emitted by things like power plants and then sequester it indefinitely in some sort of geological formation, such as a mined salt dome. While this idea is worthy of discussion in itself, my focus here is a number of approaches often described as CCS, but which do not achieve the same long-term result.
Some people have proposed that, rather than burying carbon underground, we just pump it into the sea. One option I am not going to discuss now is making big pools of liquid carbon dioxide in the very deep ocean. Rather, I will address the idea of using pipelines from shore or trailing from ships to release CO2 about 1000m down. Another alternative with similar effects is to make huge chunks of dry ice and throw them overboard, hoping most of the carbon will sink. Rather than being a type of CCS, these activities migtht be more accurately called ‘oceanic dumping of CO2.’
A matter of equilibrium
The problem here is both fundamental and intuitive. Think about a large plastic bottle of cola. With regards to the carbon dioxide, there is an equilibrium that exists between the amount dissolved in the liquid and the amount that is part of the air at the top of the bottle. As long as the system is closed (the cap is on), the amount of gas in air and water will trend towards that equilibrium point and, once the balance is achieved, stay there. This is what chemists mean when they say that equilibrium states display ‘constant macroscopic properties.’ CO2 from the water is still moving into the air, but it is now doing so at precisely the same rate as CO2 from the air is moving into the water. This is inevitable because if one rate were higher, the relative concentrations would change, and would continue doing so until the equlibrium was reached.
Now imagine that we change the equilibrium. If we take the cap off the bottle, the air inside mixes immediately with the air outside. Since the air inside has more CO2 than the air outside (because some of it has come out of the cola), this mixing causes the concentration of carbon dioxide at the surface of the cola to fall (we are ignoring the effects of atmospheric pressure in this analogy). As a consequence, the cola will start to release CO2, trying to get back to the old equilibrium between cola-dissolved and air-mixed gas. Since there is a lot more air, the equilibrium eventually reached will involve a lot less gas-in-cola. The cola goes flat. In the alternative, if we put a chip of dry ice into the cola and kept the cap on, a new equilibrium would eventually be reached in which both the cola and the air include a higher concentration of CO2.
Consequences
Dumping CO2 in the ocean thereby achieves two first-order effects. Firstly, it carbonates the sea, making it more acidic. Oceanic acidification is worrisome enough without such a helping hand. Secondly, it eventually results in an air-water balance of CO2 that is identical to the one that would have occurred if the CO2 started in the atmosphere. No matter which fluid it begins in, the same amount of CO2 at the same pressure will eventually result in the same balance between air-mixed and water-dissolved gas. It is just a matter of time. This is an important concept to understand, as it is the very heart of physical and chemical equilibria.
One big second order consequence results from this. If we do build such pipelines and do start carbonating the sea, people may decide that very carbon intensive technologies (such as coal generation or, even worse, Coal-to-Liquids) are environmentally acceptable. Using them in combination with oceanic dumping will inevitably have the same long-term atmospheric consequence as dumping the CO2 directly into the air.
Now, there is one reason for which oceanic dumping might be a good idea. Imagine there is some critical threshold for the atmospheric concentration of CO2: stay below it and things are reasonably ok, go above it and things all go wrong. In this scenario, it makes sense to store a bunch of CO2 and release it little by little. Of course, this only makes sense if we (a) only do this with CO2 we were inevitably going to release anyway (no new coal plants) and (b) aggressively cut future emissions so that the slow leak will not make us cross the threshold. Suffice it to say, this isn’t the kind of usage most advocates of CCS have in mind.